Research
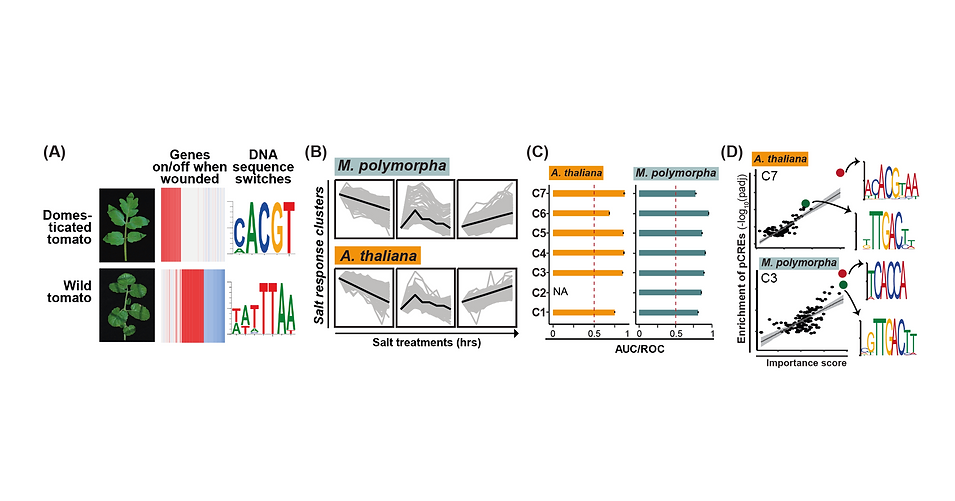
Elucidation of the transcriptional regulatory mechanisms for stress-response mRNA expressions across plants
(Liu et al., 2018, Plant Cell; Wu et al., 2021, Nature Plants)
Figure 1. The evolution of transcriptional regulatory network for plant stress responses. (A) Illustration of the evolutionary divergence of cis-regulatory elements for wounding response during tomato domestication. (B-D) The temporally salt-induced gene expression profiles (B) and the associated putative cis-elements (D) and their prediction performance (AUC/ROC) in each salt response cluster (C) in Arabidopsis and liverwort. Color dots in (D) represent top 2 cis-elements, similar to the known TF-binding motifs.
The domestication process drives the evolution of transcriptional network systems, contributing to divergent phenotypes such as stress tolerance between wild and domesticated tomato. One of the major factors contributing to plant stress tolerance is the variation in gene expression, which is controlled by the differential binding of trans-acting transcription factors on specific cis-regulatory DNA elements. Thus, to characterize the underlying regulatory mechanisms, we globally exploring stress-responsive gene expression profiles in domesticated and wild stress-tolerant tomato. We identified cis-regulatory elements, which are predictive of stress-response genes. The integration of cis-elements and transcription factor binding data further revealed the species-specific cis-elements and the likely trans-regulatory factors that control gene expression (Fig. 1A), indicating a large difference in how two these plant species turn genes on/off for stress tolerance. Our study provides new insights into how variation in gene regulatory networks contributes to variation in stress tolerance and this work has been highlighted in “IN BRIEF” in the same issue of Plant Cell. In a collaboration with Dr. Daisuke Urano’s laboratory at Temasek Life Sciences Laboratory, Singapore, we investigated how land plants evolve the gene-regulatory networks, especially the underlying trans- and cis-regulatory factors, for plant salt stress response (Fig. 1B-D). Specifically, my lab performed the cis-elements identification and gene expression prediction analyses in both the primitive (Marchantia) and flowering plant (Arabidopsis) models (Fig. 1C,D). We found that the gene regulatory networks have strictly conserved trans-regulatory factors and divergent cis-regulatory elements among different land plants. The strategy of acquiring an evolutionarily conserved trans-regulatory network to cope with the unfavorable environment and having rapidly evolving cis-regulatory elements to make the network easily evolvable may benefit land plants as they adapt to changing environments.
The discovery of hidden AUG/non-AUG translation initiation sites (TISs) and translational pausing for plant gene expressions
(Li and Liu, 2020, Genome Research; Kailasam et. al., 2020, New Phytologist)

Figure 2. The translational regulatory mechanisms at the steps of ribosome initiation and pausing. (A,B) Illustration of canonical (black arrow; A) and non-canonical (orange arrows; B) translation initiation sites (TISs). (C) The enrichments of AUG and near-cognate codons in Arabidopsis and tomato non-canonical TISs. (D) A GUG TIS initiated the translation of a small peptide. (E) A ACG TIS encodes a mitochondria-localized protein. (F) Ribosomes stall (black arrows) around the translation stop sites in the absence of NRF1.
Translation initiation determines when and where translation events start, which is crucial for protein expression. To better understand the coding diversity of plant genomes, we developed a technique of globally profiling in vivo translation initiating ribosomes and identified initiation sites on mRNAs (Fig. 2A,B). Beyond the genes identified from in silico annotation, we demonstrated the prevalent and non-canonical (i.e., unannotated) AUG and non-AUG initiation sites in both tomato (an economically important crop) and Arabidopsis (the most well-studied plant species) (Fig. 2B,C), which can lead to novel peptides or regulate protein expressions/localizations (Fig. 2D,E). We further characterized the evolutionary constraint and flexibility of translational initiation mechanisms between plants and humans. Our study and the techniques established revealed how hidden initiation sites expand the plant coding diversity and importantly will facilitate the discovery of the functional/regulatory genes, especially for the information-poor crop species.
In addition, we also collaborated with Dr. Kuo-Chen Yeh in ABRC, Academia Sinica to investigate to what extent the translational control is involved in gene expression for Fe deficiency responses. We demonstrated that an Arabidopsis gene named NON-RESPONSE TO FE-DEFICIENCY1 (NRF1) can function in proper termination of protein synthesis and also regulate plant growth and response to Fe-starvation. Specifically, my lab performed bioinformatics analyses of ribosome profiling datasets to reveal that NRF1 influenced the accumulation of ribosomes around translation termination sites (Fig. 2F). This study sheds light on NRF1-involved translation termination mechanisms and its importance in maintaining both plant molecular and physiological responses to Fe-starvation.
Revealing the secrets of a plant viral genome: new starts for protein synthesis in tomato yellow leaf curl Thailand virus (TYLCTHV)
(Chiu et. al., 2022, Plant Cell)

Figure 3. Characterization of hidden genes required for the TYLCTHV pathogenesis. (A) The translation initiation profiling in the TYLCTHV-infected tomato. (B-D) The TIS signals (B), protein evidence (C), the subcellular localizations (D) and the phenotypes of the mutates of novel TIS (blue arrows)-initiated AV2 protein isoforms. (E) Loss of novel TISs attenuated the disease symptom development. (F-I) As described in B-E, but for the novel BV2 ORF initiated at two novel TIS sites (red arrows; F).
When viruses such as begomoviruses infect plants, they hijack host translation machineries to drive expression of their proteins. This process causes viral disease and great yield losses in crops such as tomato. To gain insight into the pathogenic mechanisms, we investigated the start sites on viral mRNAs of TYLCTHV by mapping in vivo translating ribosomes (Fig. 3A). We found multiple initiation sites that were previously uncharacterized and encode new viral factors important for disease symptom development. Specifically, two downstream in-frame TISs were identified in viral protein AV2 that led to translation of different protein isoforms having different subcellular localizations (Fig. 3B-D). In addition, we characterized an unexplored gene, BV2. The BV2 protein was conserved among TYLCTHV isolates and localized in the ER and plasmodesmata (Fig. 3F-H). Mutations of AV2 isoforms and BV2 resulted in significantly attenuated disease symptoms (Fig. 3E,I). In conclusion, our study provides the first global assessment of in vivo TISs in a plant virus and also in-depth experimental investigation of novel viral proteins at the molecular and biological levels. Our findings further suggest that the complexity and diversity of plant virus coding capacity are underappreciated and this work has been highlighted in “IN BRIEF” in the same issue of Plant Cell.